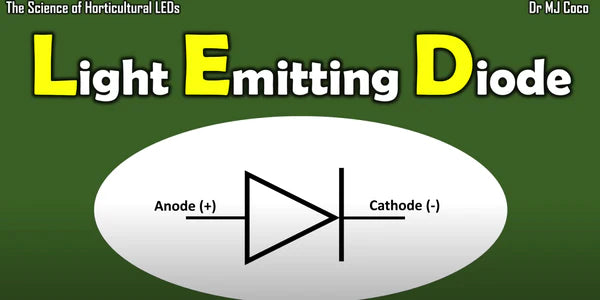
The Science of Horticultural LEDs (Mccree Curve Explained)
Over the last decade, we've gone through a technological revolution in horticultural lighting. LEDs have replaced gas discharge lights. It's been a great time to be a grower, but there's a catch.
Much of the folk wisdom about lights in the indoor growing community is no longer valid. This has created room for speculative theories and bro science and unscrupulous manufacturers have taken advantage with deceptive marketing, but it's nothing a little knowledge can't cure.
Hello growers. I'm Dr. MJ Coco from coco for cannabis.com. I created our grow light guide to help growers learn about and master horticultural lighting, and I do par and par tests to provide growers with independent data about popular lights. In this video, I dive into the science of horticultural LEDs.
I'll explain the physics of light emitting diodes and review the horticultural science about spectrum photosynthesis and plant shape. If you have questions about horticultural LEDs, I hope I'm about to answer them.
I'll cover: what is an LED. How do LEDs work? What determines the color or spectrum of LEDs? What spectrum do we want for horticulture? How do they make full spectrum LEDs? What determines how efficient LEDs are? How important is the diode brand and what else should growers know about LEDs?
I reached out to LED manufacturers for industry insight. For this video, I'd like to thank Tony and Sheldon from LEDESTAR who agreed to collaborate and have been very helpful. They provided key data, answered all of my questions, and offered valuable insight for this video.
They also provided me with some props here. As always, I retained full editorial control over my content. The analysis and advice are my own and I'm solely responsible for any errors or omissions. I'll start with the most basic question.
What is an LED? LED stands for light emitting diode. A diode is a simple semiconductor device. Diodes have a positive end, the anode, and a negative end the cathode.
This is the symbol for a diode for an LED. We add two arrows here that represent light when current is driven from the anode to the cathode in an LED light is produced in a process called electroluminescence.
More on that in a minute. Tony from Ledestar gave me this diode sample board. We can see the range of different horticultural diodes. On the left, these are all full spectrum diodes. When they're off, they look yellow because there's phosphor in the glue, but when you turn them on, they're white.
The number here describes the shape of the diode. 2835 means these diodes are 2.8 millimeters by 3.5 millimeters. 3030 is three millimeters on each side, which is a popular size for grow lights.
The other number here is the color temperature. If you look at the 3000 K and the 5,000 K, you should see a slight difference. 3000 K is warmer with more red. 5,000 K is cooler with more blue. These diodes have plastic bases and are rated for up to one wat they come from the manufacturer in a strip like this. Let me pop one out. This diode is similar to a lot of the diodes used on grow lights later in the video I'll compare the stats from this Ledestar 302H diode to the popular Samsung LM301H diodes.

Over on the other side of my sample board, they have the deep red 660 nanometer diodes. As I'll show you, crow height fixtures need 660 nanometer diodes to get the best spectrum for horticulture.
660 nanometer diodes are often higher powered and have ceramic bases like these Ledestar ceramic 3030 660 nanometer diodes. I have some of them loose. They have a clear lens on top rather than yellow glue. These 3030 are rated for up to about three watts and the ceramic 3535 red 660 nanometer diodes are rated for up to five watts.
Some grow lights will also have infrared diodes, 730 nanometers. These appear dimmer because 730 nanometer light is just at the edge of what we're able to see, and grow lights might have UVA diodes around 395 nanometers. UVB diodes at 310 nanometers are less common. I don't recommend them.
I'll explain why I'm not a fan of using UV and grow lights, and I'll discuss the spectrum that we do want for horticulture and how UV and IR fit into that. But first, let's look inside a diode at the components. I'm going to use an animation that ledestar provided.
It shows the components and the two most common types of horticultural diodes, a 3030 white full spectrum diode and a 3535 deep red 660 nanometer diode. Most LEDs on grow lights are now full spectrum white diodes rated for about 1 wat like this, 3030 white ledestar diodes.
They usually have a plastic frame with a reflective cavity. Ledestar and other good horticultural diode manufacturers we use gold foil to line the cavity. The wiring on horticultural diodes is also often gold. Gold is less reactive and prevents corrosion, particularly from sulfur.
The most important component in an LED is the semiconductor or chip. Most diodes have one semiconductor. The ledestar diode shown here has two. The semiconductors are mounted to the frame and covered in glue as I'll explain.
White diodes have phosphor mixed in with the glue. In addition to the white diodes, most grow lights will also have six 16 nanometer red diodes. This is the other diode shown. Oftentimes these diodes will be higher powered. This ledestar 3535 deep red diode is rated for up to five watts.
Higher powered diodes typically have ceramic frames which handle and dissipate heat better. Again, they use gold wiring and there's always a semiconductor. In this case, it's a six 60 nanometer semiconductor. Single wavelength diodes may use glue as a cover, but since they do not have to filter the light, they can also use a clear lens like the lead star six 60 nanometer diode here.
Okay, so that's what a horticultural LE is and what they're made of. But the most interesting question is how do LEDs work? How do they actually create light? We'll need to review some physics in order to answer this question. It's fascinating stuff, but don't worry. I'll try to keep it simple.
And it has to do with semiconductors, which are actually simpler than they sound. A semiconductor is just material that doesn't conduct electricity well but is somewhat conductive. There are natural semiconductors, but for LEDs, they use manufactured materials. LEDs use PN type semiconductors. They have P-type material fused with N-type material. Light is created as electrons cross the PN junction.
Both P and N type material are made with a non-conductive base material, usually gallium arsenide. They then add impurities in a process known as doping. The doping process makes the P and N material semi conductive but in different ways. For P type material, they add an element that is one electron short of completing its valance band.
This creates a hole where a traveling electron can pass through the holes create a positive charge, which is why it's called P type material. For N type material, they add an element that has only one electron in its valance band.
This electron can be exchanged, which allows current to pass through. The extra electron has a negative charge, hence the name N type material. When a diode is placed into a complete circuit and a forward current is applied, the free electrons and the N type material are repelled by the negative electrode and move toward the PN junction.
Electrons in the P type material also move toward the anode that makes the holes in the P type material appear to move toward the PN junction. When free electrons in the N type material reach the P type material, they need to find a hole. But here's the catch. In order to occupy that hole, the electron must move to a lower orbital level and that's what creates light.
Let's look at this even closer. And in atom, there's a nucleus that has a positive charge from the protons. Electrons orbit the nucleus. In discreet energy bands, the number of electrons that can occupy each band is limited in the first energy band, only two electrons can exist. In the second band, there can be up to eight electrons. In the third energy band, there can be up to 18 electrons. In the fourth energy band, there can be 32 electrons, and so forth.
The amount of energy that each electron has is determined by the energy band. At the lower energy bands closer to the nucleus, electrons have less energy at the higher bands. Further from the nucleus, electrons have the most energy. The outermost energy level that is occupied by electrons in a given atom is called the valance band. Electrons that are lost, gained or shared occupy the valance band and an atom is the most stable when the valance band is completely occupied.
So, in a semiconductor, we use P and N type materials. When we talk about the N type material being doped with atoms that have an extra electron, we mean that it's an atom with only one electron in the valance band. And when we talk about the holes and the P type material, we mean that it's been doped with an element that has one spot open for an electron in the valance band. LEDs create light because the open spot in the P type material is at a lower energy level than the free electron in the N type material.
In this example, it would not take much energy to knock this electron free from this N type atom, and this P type atom would be happy to receive the extra electron and complete its valance band. But this electron is coming from energy level three and the spot available to it is in energy level two. So, when we push it into that hole, energy is released. Semiconductors for LEDs are designed to release the maximum amount of this energy as light.
Depending on the specific semiconductor materials, it could be blue light, green light, red light, or even light outside the visible spectrum.
It's time for our next question.
What determines the color or spectrum of LEDs?
As I just explained, the semiconductor in an LED creates photons as electrons drop to lower orbits. If the drop in orbital energy is small, then the photon will have little energy. It'll be a long wavelength and the light will be red or far red. If the drop in orbital energy is larger, then the photon will have more energy and a shorter wavelength. The light can be blue or even ultraviolet. So, it's all about how far the electrons are forced to drop as they cross the PN injunction in a semiconductor. In theoretical physics, this distance is called the forbidden gap. You can think about it this way.
I'll put increasing band energy on a vertical access. The free electrons in the N type material are in the conduction band. They have relatively high energy. The holes in the P type material are in the valance band, they have less energy. Electrons can exist at the conduction band level of energy or at the valance band level of energy, but those are the only options for electrons passing through a semiconductor. The laws of physics dictate that electrons are not allowed to exist in the forbidden gap, that's why it's called the forbidden gap.
So, when an electron makes the jump from conduction band to valence band energy, it's instantaneous and the energy is released in the form of a photon. If the forbidden gap is small, then the drop in orbit is small and the photon produced will have little energy and a long wavelength. For example, six 60 nanometer deep red. If the forbidden gap is larger, then the photon will have more energy and a shorter wavelength like four 50 nanometer blue.
So, the size of the forbidden gap determines the wavelength of the photons that are produced, and the forbidden gap is determined by the specific doping agents used in the P and N type material. Whatever the case, each semiconductor produces a single wavelength of photons. In other words, monochromatic light. A four 50 nanometer diode uses a semiconductor that creates four 50 nanometer photons and a six 60 nanometer diode uses a semiconductor that creates six 60 nanometer photons.
There are now semiconductors that create a huge range of specific wavelengths, but each semiconductor can only create one wavelength. They all create monochromatic light. A semiconductor cannot make full spectrum or white light.
Of course, we now have full spectrum white diodes, but before I consider them, let's consider our next question.
What spectrum do we want? For horticulture? There are actually two properties of light flux and spectrum. As physicists say, light is both a particle and a wave, and as growers, we need to understand both.
Flux considers light as a particle photons. In horticulture, we measure the photosynthetic photon flux PPF, which is the number of power photons, 400 to 700 nanometers, and we measure the photosynthetic photon flux density, PPFD, which is the density of par photons at a particular spot. My par test videos are primarily about flux.
However, spectrum is also important. Spectrum considers light as a wave. Different photons have different wavelengths and different wavelengths can infect plants in several ways. As growers, we should be aware of two ways that light affects plants.
First light is the power that drives photosynthesis, and second light can also affect how the plant grows and develops.
I'll consider photosynthesis first. Photosynthesis is powered by a range of wavelengths of light. Dr. Keith McCree used data from various plants and lights to define the average photosynthetic response from different spectra of light. The result is the famous McCree curve. McCree defined the range of photosynthetically active radiation par to be 400 to 700 nanometers.
This is approximately the same range as visible light. A few important points about this curve. First, 400 and 700 are arbitrary cutoff points. It's not like a 400 nanometer photon contributes fully to photosynthesis, and the 399 nanometer photon contributes nothing.
There's a gradual tapering. McQueen knew this but was limited by the lighting technology of his day. Second, advances with LEDs and lasers have allowed more recent research to refine the range of photosynthetically active radiation. Importantly, many now argue that the 700 limits should be moved to 750. This is the new ePar that Dr. Bruce Bubby and others are advocating for.
Finally, the photosynthetic response to changing spectrum within the par range is very small. Red light between 60 and 660 nanometers is among the most efficient light for photosynthesis, but it's only about 15% more efficient than blue light for photosynthesis within the EPA range. The spectrum of light has less impact than many growers assume.
However, numerous studies have shown that monochromatic light is not efficient for horticulture, there are two subsystems involved in photosynthesis. Photo system one and photo system two. In photo system one chlorophyll A 700 is the active reaction center. It reacts primarily with longer wavelength red light. In photo system two chlorophyll A 680 is the active reaction center. It reacts primarily with shorter wavelength light.
Therefore, if you use only one wavelength, you will not activate all of the possible photosynthesis and this could cause bigger problems than just slow growth. The engineers who designed the early generations of horticultural LED fixtures recognized the need to activate both photo systems, so they included red and blue monochromatic diodes.
This produced a garish red and blue light that growers call blurple. It worked reasonably well for driving photosynthesis, but it was visually awful and makes it difficult to see the plants clearly.
Purple light has only red and blue light. The spectral output curve looks like this. Some thought that purple light was the most efficient powering photosynthesis. Many grow light companies promoted this logic, and a lot of growers started to believe that green light does not power photosynthesis.
But the truth is that purple lights were made because the red and blue diodes were more efficient and cheaper than the full spectrum diodes at the time. And despite what some growers now think green and yellow photons from 500 to 600 nanometers do power photosynthesis and they can penetrate into leaves further than red or blue photons. So, the energy in green light is not lost, and there are real advantages to having full spectrum light.
For example, you can see the plants better under full spectrum light growers. Horticultural scientist’s grow light companies and diode manufacturers all now agree that for photosynthesis, a full spectrum light covering 400 to 750 nanometers with peaks in the red and blue is the most efficient. This is the spectral output that almost all horticultural lighting fixtures now produce. To achieve it, they use a combination of full spectrum diodes and 660 nanometer red diodes.
They may include a few other diodes, but the full spectrum diodes in the 660s are the most important for photosynthesis. But beyond photosynthesis, what about the developmental impacts of spectrum? Generally in horticulture, when we alter the spectrum, we're not trying to affect photosynthesis. Rather, we're trying to change the plant shape, different wavelengths of light and signal plants to grow in different ways. We know that blue light inhibits cell expansion. Increasing the ratio of blue light during vegetative growth will produce smaller plants.
Conversely, we know that far red light enhances cell expansion. Adding far red during sprouting can help encourage early growth, but too much far red during vegetative growth may create undesirable stretch on cannabis plants during vegetation. Changing spectrum affects plant shape.
However, during flowering changing spectrum has little to no impact. Many growers think that there's some magical spectrum that will produce the best cannabinoids, but the evidence we have suggests that's not true.
For example, in research reviewed by Bug bee altering the ratio of blue light from four to 20% during the flowering period had no impact on yield or the production of cannabinoids.
You may be asking, what about infrared or ultraviolet? Many cannabis growers believe that infrared or ultraviolet light will have a positive impact on harvest quality. Some manufacturers are marketing to these beliefs, but they are not based on science.
Now, as I mentioned, far red light will contribute to photosynthesis and that could lead to larger yields. But the best research on UV light suggests that it is usually detrimental to cannabis harvest quality. I neither use nor recommend supplemental UV light for indoor growers.
For horticulture, we don't need UV, but we do want a full spectrum light covering 400 to 750 nanometers. However, as I explained, semiconductors create monochromatic light. There are no semiconductors that create white light. White light is a combination of several spectra and any given semiconductor can only create one.
So you may be asking your next question, how do they make full spectrum LEDs? There are two ways you can embed three semiconductors in a red, green, blue set, or you can use a blue semiconductor and filter the light with yellow phosphor. The second option is how the full spectrum diodes on your grow light are made. Full spectrum diodes like the Samsung LM 301H or the Ledestar 302H use semiconductors that produce 450 to 460 nanometer light.
The light that comes out of the semiconductors is blue, but then there's phosphor and other elements added to the glue or epoxy that covers the semiconductor. The phosphor filters the light and distributes the spectral output. The specific blend of phosphor and other elements will determine the color temperature of the complete diode. And of course, when you filter light, you lose some in the process. So the specific blends of phosphor that different manufacturers use can affect diode efficiency. However, phosphor is only one of many factors that affect the efficiency of LEDs. You may wonder what the other factors are.

This is our next question. What determines how efficient LEDs are? When we talk about LED efficiency or efficacy, we're thinking about light as particles, photons, and we measure the photon efficacy or the energy efficiency.
Photon efficacy PE is the number of photons in micromoles divided by the amount of energy used to create them in jewels. In horticulture, we typically focus on photosynthetic photon efficacy. PPE, the number of photons within the par range, 400 to 700 nanometers in micromoles divided by the amount of energy used to create them in jewels.
Photon efficacy and photosynthetic photon efficacy are expressed as micromoles per jewel. As we discussed, each photon within the par range contributes about the same to photosynthesis, so we just count them. However, in physics, we understand that different photons have different amounts of energy. The amount of energy is proportional to their wavelength. Shorter wavelength photons have more energy than longer wavelength photons, for example, there's more energy in a blue photon than a red photon.
So, to measure energy efficiency, we need to account for the amount of energy in the photons. Energy efficiency is the amount of energy in the photons divided by the amount of energy used to create them. Energy efficiency is expressed as a decimal or percentage as growers, we don't often have to think about this level of energy efficiency, but it will be helpful to fully understand LEDs. The two levels of LED efficiency the growers should consider are the efficiency of the individual diodes and the efficiency of the grow light fixture as a whole.
In terms of the individual diodes, there are several factors that affect efficiency, but by far the most important is the semiconductor.
In the last several decades, advancements in semiconductor materials have LED to significant efficiency gains, but in the last few years, those gains have tapered off.
Most semiconductors are now very efficient and there's little difference among the top brands. However, there are significant differences in the efficiency of generating different wavelengths.
Let's compare common horticultural diodes and think about the average efficiencies across the industry. Based on the current state of semiconductor technology. The blue four 50 nanometer semiconductors are the most efficient. They now get an energy efficiency of between 85 and 90%, so for every 10 watts of energy spent, you get up to nine watts of blue light energy. There's not a lot of room left to improve.
Blue photons have a lot of energy, so 85 to 90% efficiency translates to a photon efficacy of about 3.4 micromoles per jewel. Since the blue semiconductors are the most energy efficient, they're the base for the full spectrum diodes. However, the phosphor and the full spectrum diodes drops the efficiency slightly.
The best full spectrum diodes are now above 80% efficient, and they have photon efficacy up to about 3.1 micromoles per jewel. For comparison, HPS bulbs have an energy efficiency of only about 40% and a photon efficacy of up to about 1.6 micromoles per jewel. Now, the red 660 nanometer semiconductors are not as efficient as the blue 450 nanometer semiconductors.
In terms of energy conversion, the best six sixties now are between 70 and 75% efficient. However, compared to the blue450s, the red 660 s actually have higher photon efficacy. The 660 nanometers semiconductors can get up to 4.1 micromoles per jewel. They have more photons per jewel despite having less energy efficiency, and this is because red photons have less energy than blue photons.
The 730 nanometer semiconductors are even less energy efficient. They get between 65 and 70% efficiency, but 730 nanometer photons have even less energy, so there are actually more of them. The 730 nanometer semiconductors can get up to 4.2 micromoles per jewel. So, even though the red diodes are less efficient in terms of energy conversion, they're super-efficient for horticulture. Photosynthesis responds the best to red light, and we can make a lot of red photons with little energy using 660 or 730 nanometer semiconductors.
Down at the other end of the spectrum, you'll see that the UV semiconductors offer us much less UVA semiconductors at about 395 nanometers are only about 60 to 65% energy efficient. That might seem similar to the 730 nanometer semiconductors.
However, the photon efficacy for UVA semiconductors is only about 1.8 micromoles per jewel, so, even with a similar energy efficiency as the far red semiconductors, the UVA semiconductors produce less than half the number of photons.
This is possible because each 395 nanometer photon packs a lot of energy. As we go to shorter wavelength semiconductors, the efficiency plummets UVB semiconductors at 310 nanometers have an energy efficiency of only about 5%.
In February, 2022, the research team of Con Atall published new record setting results by improving the distribution of the doping element in the P type material, they were able to achieve 9.5% efficiency with a three 10 nanometer semiconductor.
The energy efficiency is just terrible, and because shorter wavelength photons have so much more energy, the photon efficacy of 310 nanometer semiconductors is vanishingly small, no more than 0.2 micromoles per jewel.
Now, it's possible that further research and improvements in semiconductor materials will improve these numbers, but at this point, it's far more efficient to get UV light from a fluorescent tube. If you want to use UV light despite my warnings, get a fluorescent tube and be careful.
Besides the semiconductor itself, the other components will have a minor impact on efficiency. The next most important component is the frame. Plastic frame is always cheaper, but a ceramic frame will dissipate the heat better, and since they stay cooler, dials of the ceramic frame are more efficient. The glue and the phosphor in the glue will have some impact on efficiency, as will the quality of the wiring.
Due to small differences in components and assembly, there's quite a bit of variability in the performance of the finished diodes. Manufacturers test and sort each diode into different bins.
Diode models may have two to four different efficiency bins. The top end diodes perform the best and maybe 0.2 to 0.5 micromoles per jewel more efficient than the next highest bin.
Of course, lower bin diodes are cheaper than top bin diodes, so there's a real temptation for some grow leg companies to use them. They then tout the brand and model, but do not disclose that they use a lower bin diode.
Getting the full specs about the diodes from grow leg companies can be tough. This is one of the reasons that I test fixtures, and even if you have top in diodes, your grow light still may not be efficient.
One of the biggest things that will impact the efficiency of a diode is how much power you push through it. Diodes themselves can be rated for as little as 0.2 watts or as high as a hundred watts.
For horticultural lighting, the most common full spectrum diodes have plastic bases and are rated for one watt, but the 660 nanometer and 730 nanometer diodes are often higher powered five watt diodes with ceramic bases.
However, with all diodes, if you run them at their max wattage, they're much less efficient. Ledestar provided me with data at different power levels for their 302 H diode.
Like most full spectrum diodes on grow lights, the LEDESTAR 302H is rated for 1 watt, however, you get the best efficiency at less than 20%, the maximum power with 65 milliamps at 2.72 volts, which is 0.18 watts. The LEDESTAR 302 H diode has a photosynthetic photon efficacy of 3.10 micromoles per jewel.
LEDESTAR recommends driving the 302H diodes at about 100 milliamps at 2.78 volts, that 0.28 watts a little over one quarter of the rated power capacity. The photon efficacy at this power level is still fantastic. 3.07 micromoles per jewel, they increase the power to 150 milliamps at 2.84 volts, that 0.43 watts or 43% of the rated capacity at this power level, the PPE dropped to 2.93 micromoles per jewel.
Then they increased the power to 300 milliamps at 3.02 volts. They were driving 0.91 watts of energy through the diode. This is 91% of the rated capacity at this power level. The PPE dropped to 2.57 micromoles per jewel in terms of relative efficiency. With the 65 milliamp test being the benchmark going to a hundred milliamps only lowered the efficiency to 3.07, which is 99% of 3.1. At 150 milliamps, the efficiency starts to drop. 2.93 is only 95% of the maximum and going to 300 milliamps push the efficiency down to 83%.
So, overpowering the diodes leads to considerable drops in efficiency, even with a really high quality diode. Most full spectrum diodes on grow lights are rated for one watt, but you want to run them at about a quarter to a third of a wat. This is something to consider when buying a grow light.
The number of diodes per wat is a key factor that determines fixture efficiency. On a grow light for every watt of power, you want there to be three to four full spectrum diodes. If you're buying 150 watt grow light, you want to see 450 to 600 full spectrum diodes.
Many grow like companies will use big name diodes but scrimp on diode count. The result is a fixture that markets well but performs poorly. In many cases, fixtures with lower rated diodes, but higher diode counts will be more efficient and less expensive than fixtures with top end diodes that are overpowered.
This brings us to the next question. Considering everything I've already covered, how important is the diode brand? Many growers rely on the brand name of the diodes to be confident that they're getting an efficient fixture.
However, diode brand is not a good predictor of fixture quality or efficiency. It's important to have good diodes, but there are a lot of brands that make good diodes. Many growers consider the Samsung LM 301h diodes to be the best. There are awesome diodes, but there are several other brands that have models with identical or even slightly better performance.
Let's compare the Samsung LM 3 0 1 H to the LEDESTAR 302 H diodes.
Ledestar shared their in-house test data using an ever fine Haas 2000 integrating sphere 10 diodes from both brands were tested at a current of 65 milliamps with about 2.7 volts.
That's an average of 0.177 watts per diode for both brands in 10 tests, the Samsung LM 3 0 1 H diodes had an average PPF output of 0.545 micromoles per second, which is 3.072 micromoles per jewel. The Ledestar 302H diodes had slightly higher averages across their 10 tests. The PPF output was 0.548 micromoles per second, which is 3.095 micromoles per jewel.
So, in terms of flux output and efficiency, there really is no difference between the Samsung LM301H and the Ledestar 302H. But in terms of cost, there's a considerable difference.
Samsung LM301H diodes are the most popular model and that has driven their cost up. Light manufacturers now pay about 6 cents per diode. The LEDESTAR 3 0 2H diodes are less than half. That cost light manufacturers pay only about two and a half cents per diode.
There are simple economics at play for light manufacturers. If they choose the ledestar diodes, they can afford to put a decent number of diodes into each light, but growers demand Samsung diodes.
So, many light manufacturers go with them, but then shrimp on the number in this way, the collective preference of growers for one brand of diode is actually leading to lower quality lights. Now, maybe you think the color or spectrum is different between the Samsung and Ledestar diodes.
Both sets of diodes were labeled as having a combined color temperature of 4,000. Calvin. The Samsung diodes tested in an average color temperature of 4,026 Calvin. The ledestar diodes averaged 4,053 Calvin. On the human scale 4,000 Calvin is between daylight and soft white. It's pleasant to work under and shows true colors unlike blurple light.
In terms of spectral output, the two brands of diodes are also extremely similar. They both have strong peaks in the blue troughs at about 500 nanometers and a secondary peak in the red.
The peak in the blue is due to the fact that the semiconductors put out four 50 nanometer light. The secondary peak in the red is the result of the phosphor.
Let's look back at the McCree curve. This shows how plants respond to different levels of light. There are certainly similarities to the diode output curves with two peaks, but according to the McCree curve, the plants prefer more red light.
This is why most grow lights use both full spectrum diodes and deep red six 60 nanometer diodes. The 660 nanometer diodes are very efficient and they complement the white full spectrum light.
Samsung makes 660 nanometer diodes, but most growers consider the Austria 660 nanometer diodes to be the best. Austria certainly makes a great 660 nanometer diode.
However, they have several versions and bin ratings and the efficiency varies considerably. If you find them on a cheaper fake tier, they're probably the lower rated version. The top rated OEM diodes are among the best, but other brands have diodes that can compete.
Let's compare the OSRAM six 16 nanometer 4.24 V nine diode to the lettuce star six 60 nanometer 3 51VL nine diode. These are both top bin ceramic diodes that are rated for up to five watts.
They were tested at 700 milliamps at 2.06 volts, which is 1.44 watts. This is about 29% of the rated capacity across 20 tests. The Austria’s diodes put out an average PPF of 5.845 micromoles, which is 4.06 micromoles per jewel. I told you the 660 were efficient across 20 tests.
The Ledestar diodes did ever so slightly better. They put out an average PPF of 5.935 micromoles, which is 4.13 micromoles per jewel. So, again the diodes that growers consider to be the best are great, but there are a lot of great diodes, and again, the popular brands have inflated prices. Grow light manufacturers pay over 60 cents for each of these Osram diodes. Well, they can get the lettuce star diodes for about 50 cents each.
So, maybe diode brand does matter, but not in the way most growers think.
That's one of the lessons I hope you come away from this video with, but before I wrap it up, there are a few other things that I think growers should know about LEDs.
- First, LEDs are the present and future of horticultural lighting. Guest discharge technologies like HPS and metal halide are the past.
- LEDs are now more efficient, flexible, and more precise. We can create tailor made spectral outputs and efficiently distribute dense light across the growing area.
- Second photons are photons. They are particles that carry energy but have no mass. The energy is proportional to their frequency or wavelength. Red photons have less energy than blue photons, but they all have no mass and they all travel at literally the speed of light.
- Grow light companies sometimes try to pretend that their photons are more powerful than other grow light photons or that their light penetrates more.This is nonsense, whether they come from an LED, an HPS, a candle or the sun the only difference between the photons is the number of them and their wavelength or spectrum.I discuss the optimal number of photons in all of my park test videos and as discussed, we select an optimal spectrum based primarily on photos synthetic response. Cannabis is a magical plant, but don't believe the stories about magical grow lights. Photons are photons.
- Third, if you spray sulfur, be careful with your LEDs. I asked Sheldon at LEDESTAR what he thought growers should know about LEDs. The number one thing he wants you to know is that sulfur is bad for them. Sulfur can degrade the wiring in semiconductors in an LED. If sulfur gets into the semiconductor, the LED will stop working altogether. Horticultural diode manufacturers are aware that we sometimes spray sulfur, so they go to considerable lengths to fortify their diodes against it.
Sheldon sent me an entire file about their anti-sulfur measures like using gold plating and wiring on their horticultural diodes, but still, he suggests being very careful when using sulfur, and please don't get it on the LEDs.
The best practice is to physically remove LEDs from the grow space while you're applying sulfur sprays. It was striking to me that this was something that dialed manufacturers are very concerned about, and I think most growers have no idea. Spread the word, finally, if you want to know how a grow light will perform, find a good independent par or epar test.
Fundamentally, when you're buying a grow light, you're buying a par map, a well set up PAR test will show you how much light is produced overall and how it's distributed across the canopy. The statistics that grow light companies themselves publish are always meant to make their light look good.They often don't describe the performance of the actual fixture, and they rarely give you the information that you need to make an informed choice.
Original video: https://www.youtube.com/watch?v=kDO4t6Ku9SA&t=1526s